Projects
Members pursue research with direct applications in nanotechnology
The optical properties of semiconductor nanostructures are incredibly rich. We are setting up a micro-photoluminescence (micro-PL) system that will allow us to study the light emission from nanostructures. We can grown our own ZnO nanowires using a solution-based method, in collaboration with Prof. John Capobianco in the Department of Chemistry and Biochemistry.

We also use optical microresonators with embedded nanomaterials to how the presence of the material changes the properties of the microresonator and vice versa.
This research involves the synthesis, characterization and spectroscopy of lanthanide doped nanoparticles which have attracted considerable attention due to their potential application as biolabels and in biological assays. Nanoparticles doped with lanthanide ions that emit in the infrared are also attractive to the telecommunication industry. These materials are particularly robust and resistant to chemical and photo-induced degradation making them ideal for these applications.
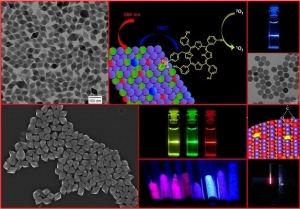
We have developed a new synthetic route based on this method that provides highly crystalline and luminescent lanthanide-doped cubic NaYF4 nanoparticles capable of being colloidally dispersed in nonpolar solvent (e.g. hexane, toluene, dichloromethane). By varying various synthetic parameters we are able to tailor the size and shape of the synthesized nanoparticles.
The synthesized particles are thoroughly characterized in terms of their physical characteristics and spectroscopic properties. Physical characterization entails powder X-ray diffraction to determine the crystalline phase and crystallite size, low and high resolution transmission electron microscopy (TEM) along with atomic force microscopy (AFM) to examine sample morphology and individual particle size, and reflectance Fourier transformed infra-red spectroscopy to detect the presence of surface species. Dynamics, luminescence efficiency and high resolution spectroscopic measurements on the dispersed nanoparticles enable us to understand the size dependence of the spectroscopic properties.
Our research is directed towards the fundamental principles involved with molecular and macromolecular 'folding'. The objectives of this research program are the design, synthesis, and physical characterization of bio-inspired model folding systems (foldamers) that adopt well-defined shapes, conformations, and functions based on the sum of weak interactions.
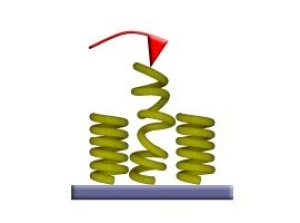
Organic synthesis is being used to access new macrocycles and helical foldamers that integrate the structural and functional characteristics of biopolymers with the stability and diversity of synthetic polymers. Scanning probe microscopies (STM and AFM) are being used to probe the self-assembly and mechanical properties of these novel materials.
Atomic force microscopy (AFM) has become a powerful tool for studying the mechanical unfolding and refolding of individual proteins and synthetic polymers. Polymeric helical foldamers are expected to have the property of being molecular-scale springs (nano-springs). We are currently using single molecule force spectroscopy to probe the physical properties of individual biopolymer molecules (chitosan) to determine their molecular-level adhesion and elastic properties.
In parallel we will perform steered molecular dynamics simulations to computationally stretch single foldamers and compare the resulting force-extension profiles with experimentally observed unfolding under external stretching forces. These experiments are yielding information into foldamer folding, unfolding, and refolding. Insight into protein folding and denaturation and in vivo mechanical stretching, thought to play a role in regulating the function of proteins polysaccharides and DNA, will be gained. Applications of these 'molecular springs' for biotechnology and nanotechnology will be thoroughly explored.
Surface interactions are ubiquitous in all aspects of life and are all controlled by weak, non-bonding interactions between molecules. Thus, knowledge of interfacial structure and the nature of interactions between surface-active components allows us to understand, predict and control interfacial processes.
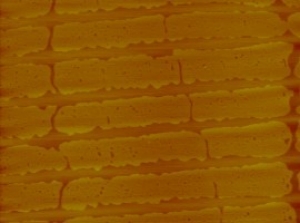
In particular, the surface of a material defines its functionality (e.g. biocompatibility, ultrahydrophobicity or electrical properties). Our research focuses on the development of surface coatings using nanopatterning techniques. We utilize a combination of self-assembly and deposition (Langmuir-Blodgett and Langmuir-Schaefer) techniques to create monomolecular films with control over chemical functionality and lateral organization on the nanometer scale. The utility of the nanopatterning approach lies in combining the morphological control of LB deposition with the versatility of SAMs for improved functionality.
The significance is in potential applications, e.g. removal of physisorbed material from a striped pattern could yield nanowires (residual stripes of conducting material) fixed to a solid substrate. The creation of multifunctional patterns and complex patterns will yield an opportunity to tailor the process to fit applications, e.g. selective biomolecule adsorption and spatially confined surface initiated polymerization. With a background in biophysical chemistry much of this work is currently directed at the design of materials with biocompatible properties and/or the ability to tether specific protein substrates. We also apply the same principles to developing surface coatings for nanoparticles in order to increase their biocompatibility while retaining the relevant physical (e.g. optical) properties. This research relies heavily on surface characterization techniques (e.g. Langmuir films, atomic force microscopy, polarized light microscopy, ellipsometry, grazing incidence x-ray diffraction, surface rheology).
Our research is focused on developing new methods for the formation of carbon-carbon bonds. This plays an important role in the synthesis of a wide range of chemical structures, including many drugs that are used to treat numerous diseases. Towards this end, we employ carboxylic acids and their mimics to create novel reactive groups that improve on the current transition-metal based coupling partners. The employment of carboxylic acids has many advantages, including their wide availability, general stability and their low environmental impact since no metal-based side-products are formed. While using sulfinate salts allow for a more facile extrusion of SO2 compared to CO2, rendering the coupling of aryl and heteroaromatic sulfinates with aryl bromides possible without the need of a co-catalyst.
My research is currently focused on studying different formulations of heterogeneous catalysts for various reaction pathways relevant for a carbon neutral future.
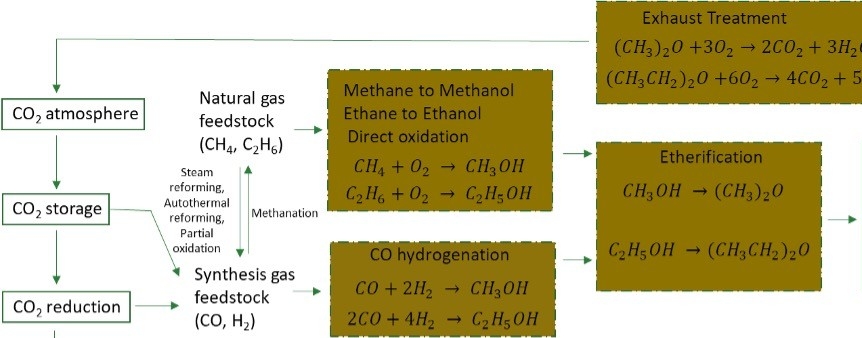
Heterogeneous catalysts generally consist of metal nanoparticles or other nano- or atomic- sized active sites supported on high surface area materials. The particular reactions being studied include CO2 hydrogenation to methanol, methanol dehydration, bio-oil upgrading, and hydrocarbon oxidation for environmental remediation. We utilize kinetic measurements on the bench scale, and in-situ spectroscopy of reactions to understand catalyst kinetics and mechanisms.
Research in the Howarth group is focused on the design of new rare-earth (RE) metal–organic frameworks (MOFs) for applications in adsorption, catalytic breakdown, and sensing of contaminants in water.

We target specific MOF topologies with structural features that we believe are useful for our application goals, creating a feedback loop involving design→synthesis→testing→design to ultimately generate champion materials. Long-term goals involve developing green MOF synthetic protocols to ensure that materials being used for environmental applications are synthesized following the principles of green chemistry.
Gold nanoparticles (AuNPs) are engineered nanomaterial having historical importance in a wide range of applications from physics and chemistry to biology and medicine. In this connection, interparticle distance-dependent surface plasmon resonance (SPR) phenomena is the reason for naked-eye readout due to changing the color of solution. The unique features of AuNPs such as their ease of preparation, diversified surface functionalization and variable morphology make these nanoparticles a primary choice for sensor fabrication. The focus of this research project is on improving the sensitivity, reproducibility, multiplexing capabilities and cost-effectiveness for colorimetric detection of various analytes of interest in environment food matrices. The progress in this filed will have significant impact on fast testing of hazardous substances, hence reducing the pollution load in environment as well as render food contamination to ensure food safety.
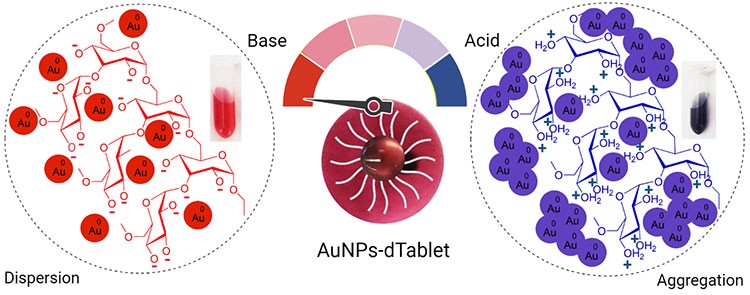
List of selected publications from 2014 to 2022
- Sadiq Z, Safiabadi Tali SH, Jahanshahi-Anbuhi S. Gold Tablets: Gold Nanoparticles Encapsulated into Dextran Tablets and Their pH-Responsive Behavior as an Easy-to-Use Platform for Multipurpose Applications. ACS Omega. 2022 Mar 22. DOI
- Safiabadi Tali SH, LeBlanc JJ, Sadiq Z, Oyewunmi OD, Camargo C, Nikpour B, Armanfard N, Sagan SM, Jahanshahi-Anbuhi S. Tools and techniques for severe acute respiratory syndrome coronavirus 2 (SARS-CoV-2)/COVID-19 detection. Clinical Microbiology Reviews. 2021 May 12;34(3):e00228-20. DOI
- Esfahani AR, Sadiq Z, Oyewunmi OD, Tali SH, Usen N, Boffito DC, Jahanshahi-Anbuhi S. Portable, stable, and sensitive assay to detect phosphate in water with gold nanoparticles (AuNPs) and dextran tablet. Analyst. 2021;146(11):3697-708. DOI
- Oyewunmi OD, Safiabadi-Tali SH, Jahanshahi-Anbuhi S. Dual-modal assay kit for the qualitative and quantitative determination of the total water hardness using a permanent marker fabricated microfluidic paper-based analytical device. Chemosensors. 2020 Dec;8(4):97. DOI
- Leung V, Mapletoft J, Zhang A, Lee A, Vahedi F, Chew M, Szewczyk A, Jahanshahi-Anbuhi S, Ang J, Cowbrough B, Miller MS. Thermal stabilization of viral vaccines in low-cost sugar films. Scientific Reports. 2019 May 21;9(1):1-1. DOI
- Jahanshahi-Anbuhi S, Kannan B, Pennings K, Ali MM, Leung V, Giang K, Wang J, White D, Li Y, Pelton RH, Brennan JD. Automating multi-step paper-based assays using integrated layering of reagents. Lab on a Chip. 2017;17(5):943-50. DOI
- Marziano V, Pugliese A, Merler S, Ajelli M. Detecting a surprisingly low transmission distance in the early phase of the 2009 influenza pandemic. Scientific reports. 2017 Sep 26;7(1):1-9. DOI
- Hsieh PY, Monsur Ali M, Tram K, Jahanshahi‐Anbuhi S, Brown CL, Brennan JD, Filipe CD, Li Y. RNA protection is effectively achieved by pullulan film formation. ChemBioChem. 2017 Mar 16;18(6):502-5. DOI
- Jahanshahi-Anbuhi S, Kannan B, Leung V, Pennings K, Liu M, Carrasquilla C, White D, Li Y, Pelton RH, Brennan JD, Filipe CD. Simple and ultrastable all-inclusive pullulan tablets for challenging bioassays. Chemical Science. 2016;7(3):2342-6. DOI
- Liu M, Hui CY, Zhang Q, Gu J, Kannan B, Jahanshahi‐Anbuhi S, Filipe CD, Brennan JD, Li Y. Target‐induced and equipment‐free DNA amplification with a simple paper device. Angewandte Chemie International Edition. 2016 Feb 18;128(8):2759-63. DOI
- Jahanshahi-Anbuhi S, Pennings K, Leung V, Kannan B, Brennan JD, Filipe CD, Pelton RH. Design rules for fluorocarbon-free omniphobic solvent barriers in paper-based devices. ACS Applied Materials & Interfaces. 2015 Nov 18;7(45):25434-40. DOI
- Kannan B, Jahanshahi-Anbuhi S, Pelton RH, Li Y, Filipe CD, Brennan JD. Printed paper sensors for serum lactate dehydrogenase using pullulan-based inks to immobilize reagents. Analytical Chemistry. 2015 Sep 15;87(18):9288-93. DOI
- Sicard C, Glen C, Aubie B, Wallace D, Jahanshahi-Anbuhi S, Pennings K, Daigger GT, Pelton R, Brennan JD, Filipe CD. Tools for water quality monitoring and mapping using paper-based sensors and cell phones. Water Research. 2015 Mar 1;70:360-9. DOI
- Jahanshahi‐Anbuhi S, Pennings K, Leung V, Liu M, Carrasquilla C, Kannan B, Li Y, Pelton R, Brennan JD, Filipe CD. Pullulan encapsulation of labile biomolecules to give stable bioassay tablets. Angewandte Chemie International Edition. 2014 Jun 10;53(24):6155-8. DOI
- Jahanshahi-Anbuhi S, Henry A, Leung V, Sicard C, Pennings K, Pelton R, Brennan JD, Filipe CD. based microfluidics with an erodible polymeric bridge giving controlled release and timed flow shutoff. Lab on a Chip. 2014;14(1):229-36. DOI
Research in Dr. Li’s group focuses on the development of nanomaterials and nanotechnologies for battery applications, such as Li-ion batteries, Na-ion batteries, metal-sulfur batteries, and solid-state batteries. The projects include the synthesis of battery materials, interface design in batteries, advanced characterization of battery materials, etc.
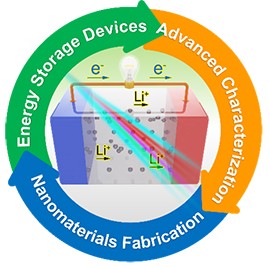
List of selected publications from 2021
- He M, Li X, Li W, Zheng M, Wang J, Ma S, Ma Y, Yin G, Zuo P, Sun X. Immobilization and kinetic promotion of polysulfides by molybdenum carbide in lithium-sulfur batteries. Chemical Engineering Journal. 2021 May 1;411:128563.
- He M, Li X, Holmes NG, Li R, Wang J, Yin G, Zuo P, Sun X. Flame-Retardant and Polysulfide-Suppressed Ether-Based Electrolytes for High-Temperature Li–S Batteries. ACS Applied Materials & Interfaces. 2021 Aug 9;13(32):38296-304.
- He M, Li X, Yang X, Wang C, Zheng ML, Li R, Zuo P, Yin G, Sun X. Realizing Solid-Phase Reaction in Li–S Batteries via Localized High-Concentration Carbonate Electrolyte. Advanced Energy Materials. 2021 Aug;11(31):2101004.
Research in the Majewski group focuses on developing, characterizing and optimizing new inorganic materials for catalyzing visible light-driven processes. Research thrusts include the development of photocatalytic metal-organic compounds and surfaces for “solar fuels” generation (i.e. water oxidation or CO2 reduction), value-added chemical transformations, and surface modification.
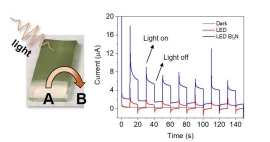
Owing to the dramatically decreased cost of solar panels (i.e. photovoltaics), in solar energy utilization, the main research opportunities that exist lie in the development of high efficiency conversion materials as well as persistent grid-scale storage to compensate for the intermittency of sunlight. Solar fuels generation, or fuel forming reactions such as water oxidation to O2 and subsequent reduction of protons to generate H2 may be carried out by homogeneous molecular catalysis or by catalysis on a heterogeneous surface. Solar H2, regardless of the source, may be used directly as a fuel, or indirectly by reaction with carbon-based feedstocks like CO2 or biomass to yield methanol or more desirable C2+ fuels. While mechanistic investigations are often easier to carry out in homogeneous systems, heterogeneous catalysts benefit from increased stability and recyclability. A marriage of the two approaches involves the adsorption of molecular chromophores and known catalysts to heterogeneous semiconductor surfaces resulting in devices where the overall energetics are well understood. An integrated photoelectrochemical system should capitalize on opportunities that lie in cohesive integration of cheap materials in new form factors and geometries coupled to earth abundant (photo)electrocatalysts. A more fundamental consideration is one of the mechanistic investigations of dye-sensitized photoelectrode architectures, where such work could lead to improved device performance.
Our work addresses the challenging problem of storing energy from the sun in chemical bonds through the development and optimization of molecular light-absorbing chromophores along with molecular chromophore-catalyst adducts, and the functionalization of semiconductor surfaces for photoelectrochemical catalytic redox processes in dye-sensitized photoelectrochemical cells (DS-PECs).
Our research aims to investigate multiple avenues in the synthesis of carbon dots in order to devise methods of preparing highly monodisperse particles with narrow size distributions and specific optical signatures with a specific focus on the experimental parameters and their impact on the nucleation and growth processes of these carbon dots.
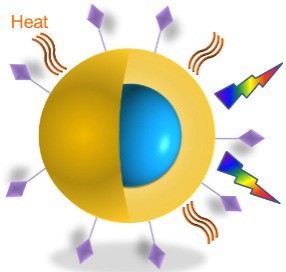
In a parallel avenue, we are also interested in the development of multiphoton excited dots, which can be used as optical probes for deep tissue imaging applications. Lastly, we also investigate core-shell systems and study synergistic interactions between metallic and luminescent nanoparticles for the design of hybrid multifunctional nanomaterials. To achieve these objectives, we rely on state-of-the-art characterization techniques such as transmission electron microscopy, x-ray photoelectron, steady-state and dynamic optical spectroscopies, to name a few. The ability to modulate the physico-optical properties of our materials will pave the way for application development in the areas of food safety and smart packaging, multiplexing assays for environmental cleanup, biomarker identification, bacterial and viral sensing, as well as imaging, diagnostics, active targeting and controlled-release drug delivery.
Our research focuses on the design, synthesis, and characterization of functional nanomaterials for photocatalysis applications. The special interests are integrating one-dimensional metal oxides with visible-light absorber materials to drive water splitting and CO2 conversion reactions.

List of selected publications from 2014 to 2021
- Nguyen NT, Xia M, Duchesne PN, Wang L, Mao C, Jelle AA, Yan T, Li P, Lu ZH, Ozin GA. Enhanced CO2 photocatalysis by indium oxide hydroxide supported on TiN@TiO2 nanotubes. Nano Letters. 2021 Jan 25;21(3):1311-9. https://dx.doi.org/10.1021/acs.nanolett.0c04008
- Nguyen NT, Yan T, Wang L, Loh JYY, Duchesne PN, Mao C, Li P, Jelle AA, Xia M, Ghoussoub M, Kherani NP, Lu ZH, Ozin GA. Plasmonic titanium nitride facilitates indium oxide CO2 photocatalysis. Small. 2020 Nov 17;16:2005754. https://doi.org/10.1002/smll.202005754
- Nguyen NT, Ozkan S, Hejazi S, Denisov N, Tomanec O, Zboril R, Schmukiu P. Providing significantly enhanced photocatalytic H2 generation using porous PtPdAg alloy nanoparticles on spaced TiO2 nanotubes. International Journal of Hydrogen Energy. 2019 Aug 30;44(41):22962–71. https://doi.org/10.1016/j.ijhydene.2019.06.200
- Nguyen NT, Ozkan S, Tomanec O, Zhou X, Zboril R, Schmuki P. Nanoporous AuPt and AuPtAg alloy co-catalysts formed by dewetting–dealloying on an ordered TiO2 nanotube surface lead to significantly enhanced photocatalytic H2 generation. Journal Materials Chemistry A. 2018 Jun 29;6:13599–606. https://doi.org/10.1039/C8TA04495C.
- Altomare M, Nguyen NT, Hejazi S, Schmuki P. A cocatalytic electron-transfer cascade site-selectively placed on TiO2 nanotubes yields enhanced photocatalytic H2 evolution. Advanced Functional Materials. 2018 Jan 10;28:1704259. https://doi.org/10.1002/adfm.201704259
- Nguyen NT, Hwang I, Kondo T, Yanagishita T, Masuda H, Schmuki P. Optimizing TiO2 nanotube morphology for enhanced photocatalytic H2 evolution using single-walled and highly ordered TiO2 nanotubes decorated with dewetted Au nanoparticles. Electrochemistry Communications. 2017 Jun;79:46-50. https://doi.org/10.1016/j.elecom.2017.04.016
- Ozkan S, Nguyen NT, Hwang I, Mazare A, Schmuki P. highly conducting spaced TiO2 nanotubes enable defined conformal coating with nanocrystalline Nb2O5 and high performance supercapacitor applications. Small 2017 Apr 11;13:1603821. https://doi.org/10.1002/smll.201603821
- Nguyen NT, Ozkan S, Hwang I, Zhou X, Schmuki P. Spaced TiO2 nanotube arrays allow for a high performance hierarchical supercapacitor structure. Journal of Materials Chemistry A. 2017 Feb 7;5:1895–901. https://doi.org/10.1039/C6TA10179H.
- Nguyen NT, Ozkan S, Hwang I, Mazare A, Schmuki P. TiO2 nanotubes with laterally spaced ordering enable optimized hierarchical structures with significantly enhanced photocatalytic H2 generation. Nanoscale. 2016 Oct 14;8:16868–73. https://doi.org/10.1039/C6NR06329B
- Nguyen NT, Altomare M, Yoo JE, Taccardi N, Schmuki P. Noble metals on anodic TiO2 nanotube mouths: thermal dewetting of minimal Pt co-catalyst loading leads to significantly enhanced photocatalytic H2 generation. Advanced Energy Materials. 2016 Jan 21;6:1501926. https://doi.org/10.1002/aenm.201501926.
- Nguyen NT, Altomare M, Yoo J, Schmuki P. Efficient photocatalytic H2 evolution: controlled dewetting–dealloying to fabricate site-selective high-activity nanoporous Au particles on highly ordered TiO2 nanotube arrays. Advanced Materials. 2015 May 27;27:3208–15. https://doi.org/10.1002/adma.201500742.
- Nguyen NT, Yoo J, Altomare M, Schmuki P. “Suspended” Pt nanoparticles over TiO2 nanotubes for enhanced photocatalytic H2 evolution. Chemical Communications. 2014 Sep 4;50:9653–6. https://doi.org/10.1039/C4CC04087B.
Our research focuses on the design and processing of macromolecular nanoscale biomaterials for biomedical applications. Our particular interests are the integration of nanostructured materials with biology and biomedicine to develop advanced bionanomaterials that can interface biological processes as well as to understand their biological functions.
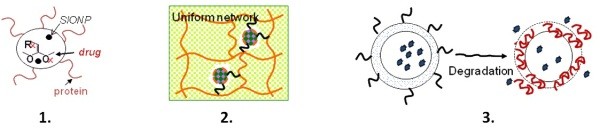
To achieve these goals, we make use of various disciplines including organic, polymer, surface, colloidal chemistry, and biomedical engineering as well as collaborate with other research groups.
As the initial thrust, our research group develops major biomaterials including novel superparamagnetic bionanogels, rapid thermoresponsive hydrogels, and nanocapsules, addressing important problems in the areas of biomedicine:
- Tumor-specific superparamagnetic acid-labile nanoscaffold bioconjugates for simultaneous MRI diagnosis and treatment of tumors in vivo
- Nano-gel attached nanoporous rapid thermoresponsive hydrogel scaffolds for tissue engineering
- Nanocaspules with biodegradable polymeric shell through degradation for drug delivery
We explore the physico-chemical properties of organic electronic materials in the solid state, where we mainly focus on thin films of organic semiconductors (conjugated molecules and polymers) with regards to their application in novel electronic devices. Such materials are of particular interest as their opto-electronic properties (e.g., their bandgap) can be widely tuned by the chemical structure and as they allow for large-area processability of functional structures.
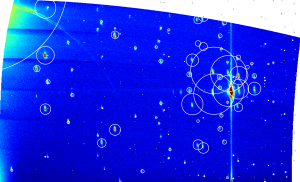
We process organic (semi)conductors from solution under inert atmosphere (via spin-coating and dip-coating) or by their thermal sublimation under ultra-high vacuum conditions into multilayer structures. Via electrospray deposition from solution into the vacuum we are further able to grow organic thin films of macromolecules and polymers in an ultrapure environment. Apart from organic electronic materials, we aim to apply our surface scientific approach to active pharmaceutical ingredients, likewise organic molecules, where their growth into surface mediated polymorphs can increase their bioavailability.
We are dedicated to exploring the structural and opto-electronic properties of these systems by employing various spectroscopic techniques and X-ray diffraction at synchrotron radiation sources. We work on deliberately modifying the electronic properties of organic semiconductors by their electrical doping, on which today the entire inorganic semiconductor technology is based, but which is still under development for this class of materials. In this field, we strive both for a better understanding of the fundamental processes involved, which seem highly dissimilar to those in inorganic systems, and for employing this understanding in organic and hybrid electronics.
The Skinner research group is developing polyelectrolyte nanocapsules to extract toxic trace metals from environmental samples. Nanocapsules have high surface area to volume ratios allowing rapid and efficient extraction of metals from solution. The highly charged surface also aids the extraction because of its high permeability to ions.
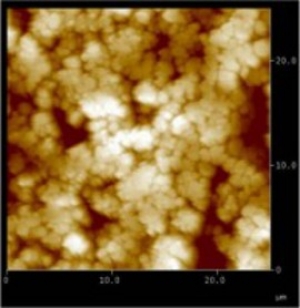
The encapsulation process is started by adding a solution of polyanions to a suspension of positively charged colloidal particles. The polyanions coat the surface of the particle completely, resulting in the reversal of the terminal surface charge. Then a solution of polycations are used to coat the newly negative surface, forming a bilayer. The thickness of the polyelectrolyte shell can be accurately controlled from 5 to 50 nm based on the number of deposited layers. After the shell is formed, the core particles are dissolved to generate hollow polyelectrolyte capsules that contain a solution of metal chelator in an organic solvent.
When the chelating capsules are suspended in a sample of water they act as scavengers for metal ions that diffuse through the capsule walls. Our current efforts are to incorporate super paramagnetic crystals within the capsule to allow the capsules to be addressable by a magnetic field that would facilitate recovery of the capsules and chelated trace metals.
Our research involves three intersecting areas: green energy via emerging 3rd generation photovoltaics, electrocatalysts, and batteries; disruptive technologies by way of optical sensors, anti-viral surfaces, and on-chip optical isolators; and advanced materials development for nanoparticles, hybrid organic-inorganic perovskites, and ferroelectrics. Our research vision is to develop easy, versatile, and inexpensive methods of producing, tuning and exploring nanostructures, targeting energy applications, sensing and magneto-optics. By making cheaper, more accessible, and more flexible products, we make an impact on how people use clean energy, access information and measure the world around them.
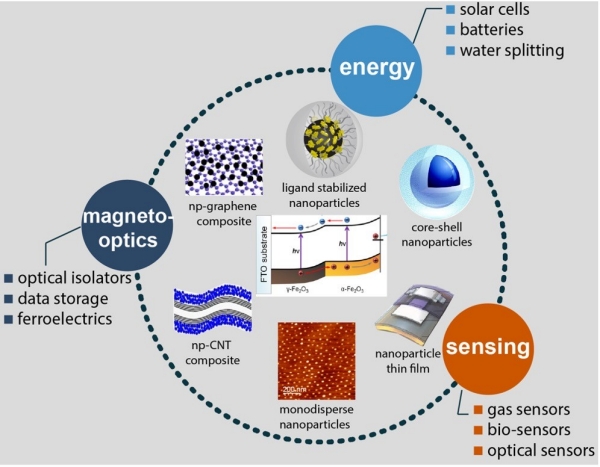
Highlighted projects
Nanostructures for disruptive applications
Projects focus on producing nanoparticle decorated and hierarchical nanostructured materials as electrodes for optoelectronic devices, batteries and fuel cells, for ferroelectric energy storage, for catalytic water splitting, as novel sensors, and for magneto-optics. We have also expanded into health technologies examining the use of anti-viral nanoparticles to combat COVID-19 and developing a new paradigm for nanoparticle enhanced radiation treatment using lithium fluoride (LiF) nanoparticles to locally enhance organic film degradation under keV X-ray irradiation at relatively low ionizing radiation doses. Highlights include highly stable organo-halide perovskites emitting across the visible spectrum; metal oxide, metal nitride, core-shell structured electrodes for efficient hydrogen and oxygen evolution; magnetic core-shell structures for optical isolation in photonics circuits; and dielectric interlayers for organic optoelectronics devices.
Spatial order in nanoparticle dispersions
Projects focus on understanding and manipulating the spatial organization of nanoparticle arrays, to go from highly ordered periodic arrays to complete spatial randomness. The spatial order of nanostructures has implications in many realms. As quantification of disorder is difficult, we have been developing a variety of tools to quantify the spatial order of 2D dispersions collected in a freely available Mathematica package, “Detecting Intermolecular Structure Located at particle positions” (dis-Locate) (available on GitHub). With the aid of such tools, we have been able to use the substrate surface energy as a parameter to tune nanoparticle dispersion and unravel the relationship between device performance and geometric order and dispersion of nanostructures. These tools are also being applied to other systems, including the organization of opals and inverse opals as photonic crystals (with Y. Salinas, Johannes Kepler University Linz, Austria), the distribution of self-trapping filaments in polymer waveguides (with K. Saravanamuttu, McMaster University), and the distribution of dopants for OLEDs (with W. Y. Kim at Hoseo University, Republic of Korea).
Characteristics and characterization of diblock copolymer reverse micelles for nanostructure templating
Projects focus on understanding the process of nanoparticle formation using reverse micelles. This includes the development and integration of modeling to understand core-shell behavior, novel characterization methods, (uc-TARS and QNM for infiltration), identification of new Raman modes to understand salt complexation, and knowledge of the synthesis process to understand the impact of changing parameters. We have developed new recipes for functional nanoparticles and explored myriad ways to modify the spacing and distribution of the resultant nanostructures.
Dr. Zhibin Ye’s research group focuses on designing and developing innovative nanostructured materials for electrochemical energy storage in supercapacitors and rechargeable batteries, catalytic organic transformations, and removal of hazardous substances in environmental applications. Some representative nanostructured materials being developed include ultra-small carbon nanospheres with well-defined diameters within 5 – 50 nm for energy storage, ionomer-modified 2D MXene materials for batteries, and graphene-based nanocomposites for arsenic removal.

My research is currently focused mainly on exploring structure-function relationships in photosynthetic pigment-protein complexes. These nano-scale objects are responsible for the first, light-driven steps of photosynthesis. The particular issues being explored include pigment-pigment and pigment-protein interactions, excitation energy transfer, as well as low-temperature dynamics of proteins. We utilize mainly the methods of optical spectroscopy, including high-resolution low-temperature methods such as spectral hole burning and single molecule/complex spectroscopy.

In terms of artificial mimics of photosynthetic complexes, I am primarily interested in the synthesis and properties of pigment-functionalized virons of Tobacco Mosaic Virus. These virons are extremely stable natural nanotubes, and various groups around the world are currently seeking to utilize them in different applications. Fluorescence peaks due to single Photosystem I complexes of cyanobacterium Synechocystis PCC6803.
On an applied side, I am interested if natural and artificial photosynthetic reaction centers could be utilized for explosives sensing. Explosives have structure and chemical properties close to those of the herbicides, which inhibit photosynthesis by disrupting electron transfer in photosynthetic reaction centers.